Targeted Endodontic Microsurgery requires dependable and efficient 3D printing. Read this study by Dr. Julie Anderson and colleagues for a comparison of some 3D printers.
Drs. Julie A. Anderson, James A. Wealleans, and Jarom J. Ray discuss safe and effective 3D printing to produce surgical stents
Introduction
Applications of three-dimensional (3D) printing appear in the endodontic literature for guided access, autotransplantation, and endodontic surgery.1-6 The majority of endodontic publications utilized patented, jetting-type printers such as PolyJet (Stratasys Ltd, Austin, Texas), MultiJet (3D Systems, Rock Hill, South Carolina), or ColorJet (3D Systems, Rock Hill, South Carolina) (Table 1).7 Jetting-type printers extrude thin layers of proprietary photopolymer material onto a build tray where the layers are cured immediately after deposition. Each subsequent layer is deposited upon the previous layer, as the build platform moves downward, away from the extruder.8 These printers have been shown to be highly accurate with minimal post-processing requirements, but their size and cost may be prohibitive to use in individual endodontic practices.8 Alternative technologies such as stereolithography apparatus (SLA) and digital light processing (DLP) printers have price points and size profiles more amenable to widespread deployment throughout endodontics.9 Both SLA and DLP printers utilize vats of photosensitive resin in which the build platforms are submerged, yet they differ in their methods of curing the resin. SLA employs a focused UV laser to trace the cure locations for the object being printed, whereas DLP utilizes a projector that exposes the entire layer simultaneously.8 In both systems, the platform is raised out of the resin vat as the build is completed from the bottom upwards. The Form 2 (FormLabs Inc, Somerville, Massachusetts) is an SLA printer costing about $3,500. The Duplicator7 (Wanhao, Zhejiang, China) is a DLP printer costing about $500 (Table 1).
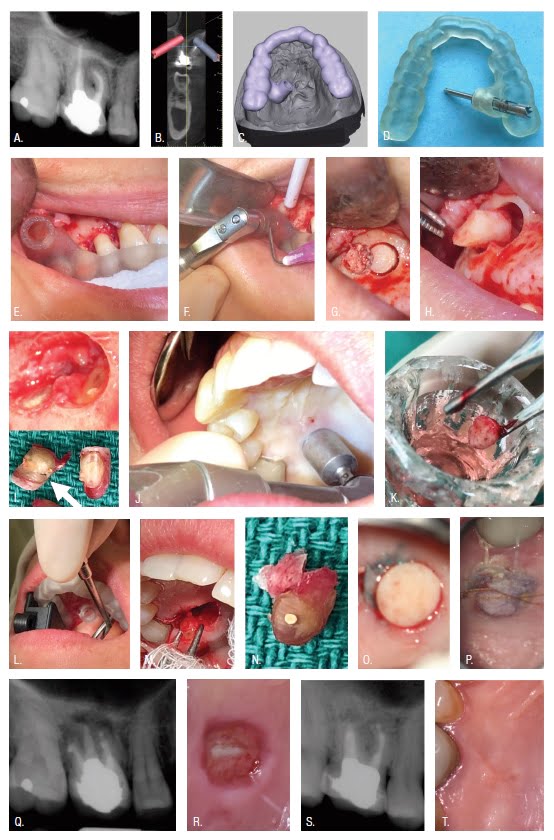
Targeted Endodontic Microsurgery (TEMS) uses 3D printing to produce surgical stents capable of guiding a trephine bur according to exacting design specifications.10 A trephine is rotated within the guide port of a stent producing osteotomy, root end resection, and biopsy in a single step. Figure 1 shows surgical access to the palatal root of the maxillary first molar (A), implant-planning software used to define the TEMS pathway for removal of palatal and facial root ends (B), guides designed to seat on the patient’s dentition (C), exacting guidance of a trephine (D), a trephine oriented by a guide seated upon the patient’s dentition (E), a trephine in position for rotation at 900-1,200 revolutions per minute (rpm) (F), osteotomy and root end resection (G,H), en bloc removal of a biopsy specimen (I), palatal soft tissue removal using a rotary biopsy punch (J), soft tissue preserved in Hank’s Balanced Salt Solution (K), trephine resection of the palatal root end (L-N), soft tissue approximated and sutured to serve as a scaffold for healing (O,P,R,T), allowing for treatment of three root ends, (Q) and healing at 1-year recall (S). The surgical guides (3DSGs) used in the introductory TEMS publication were printed with an Objet260 Connex 3 (Stratasys Ltd, Austin, Texas) PolyJet printer costing in excess of $120,000.10 To date there have been no evaluations of jetting-type, SLA, or DLP printers for TEMS or guided endodontic access.
Previous engineering studies have evaluated printer accuracy for endodontic and non-endodontic applications by using a 3D scanner and software to compare the dimensions and volume of a printed part to its virtual design.11-14 These studies provide quantification of the relative conformity of a printer’s product with the original design, but clinical acceptability is not necessarily established, as such studies do not define how much dimensional and volumetric deviation from the digital design will produce a clinically acceptable stent.12 Further, if a printer deviates from design dimensions in a consistent manner, adjustments to design can be made to render print products clinically acceptable. For example, a printer that consistently prints slightly smaller than the target dimension can be corrected for in the design phase by adjusting dimensions. The same is not true for an inconsistent printer that sometimes prints larger than design dimensions and sometimes smaller.
A gap in knowledge exists in that the clinical acceptability of individual three-dimensional printers (3DPs) for TEMS applications is unknown. The purpose of this study is to quantify conformity between design dimensions and printed objects for the Objet260, Form 2 and Duplicator7 3DPs (Part I), and then to conduct a clinically oriented assessment of the acceptability of TEMS stents produced by each of these printers (Part II). For Part I, the null hypothesis was that printed objects from all printers will precisely conform to digital design dimensions. For Part II, the null hypothesis was that all 3DSGs from the three printers will be clinically acceptable in this simulation model
Materials and Methods
Part I: Dimensional comparison
A template with five cylindrical holes of varying diameter was designed using SOLIDWORKS 2018 software. Hole diameters were chosen based on the ISO diameters of a friction grip bur (1.6 mm), a latch-type (2.35 mm), and three trephine burs (4.0 mm, 5.0 mm, and 6.0 mm). The standard tessellation language (STL) file was used to print 30 templates from each of the three printers according to manufacturer’s instructions (Figure 2A). The Objet260 required proprietary MED610 resin (Stratasys Ltd, Austin, Texas). In contrast, the Form 2 and the Duplicator7 could not use MED610, and so templates were printed with NextDent SG Resin (3D Systems, Soesterberg, Netherlands). Templates were post-processed according to manufacturer’s instructions and then scanned by a benchtop scanner (3Shape D1000; Whip Mix Corp, Louisville, Kentucky). GeoMagic Studio 2014 software was used to measure the diameters of the printed templates as in previous accuracy studies (Figure 2B).11-13,15 For all 150 cylindrical holes, the absolute difference between the printed diameter, as measured using GeoMagic Studio, and the virtual diameter, as input into SOLIDWORKS during the design phase, was measured, and the data was subjected to a univariate analysis of variance (ANOVA) and post-hoc Tukey’s test.
Part II: Clinically oriented assessment of TEMS stents
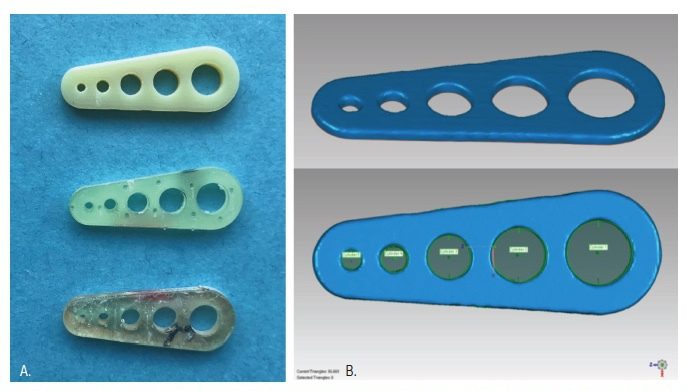
The entire digital workflow, 3D printing, and post-printing quality evaluation of the experimental model and 3DSGs were conducted by a consensus group comprised of two Board-certified endodontists, an endodontic resident, a biomedical engineer, and a medical CAD/CAM design specialist.
Experimental printed cast: Based upon accuracy data from Part I, the Objet260 was used to print the experimental model utilized during Part II. The original anonymized CBCT and STL files from Case 1 of the introductory TEMS publication for palatal root surgery of tooth No. 2 were used to print a maxillary model.10 The CBCT region of interest for the experimental model included the entire maxillary dental arch, hard palate, floor of the nose, and most of the maxillary sinus. Anatomy of the model extended 12 mm superior to the apices of the maxillary teeth. CBCT DICOM files were exported as an Axial series at 0.160 mm slice thickness. An impression of the patient’s maxillary arch was made and poured in Die-Keen stone (Kulzer, South Bend, Indiana), and then optically scanned with a 3Shape benchtop D1000 scanner (3Shape, Warren, New Jersey). Resultant DICOMs from CBCT and STL from the scanner were uploaded into Mimics design software (Materialise Medical, Plymouth, Michigan). The scan was aligned to the patients’ CBCT using tooth surface data with multiple points of reference. Within Mimics, a Medical CAD/CAM Director and a Biomedical Engineer adjusted thresholding to digitally reproduce the patient’s maxilla. Prior to printing, the trephine path dictated by the original stent design utilized in the successful surgery was applied to the model within design Mimics Innovation Suite (Materialise Medical) software to produce a 6 mm diameter cylindrical void replicating the original osteotomy and root-end resection of tooth No. 2 that occurred in the successful clinical case. Thus, a single model with a clinically ideal osteotomy reproduced the surgical scenario (Figure 3A).
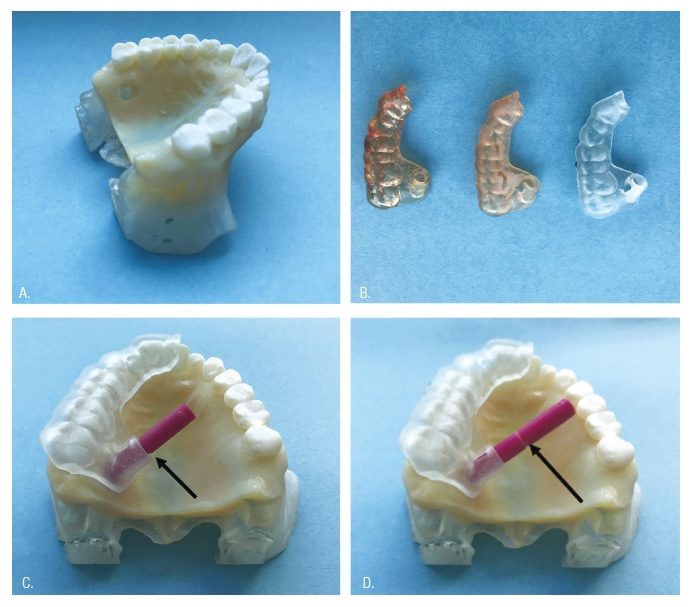
Pilot calibration: Each 3DP employs different data integration methods and printing mechanics and compatible resin. Printed products conform to design software specifications to varying degrees based upon these factors. In order to understand the performance profiles of each test printer, multiple stents were produced and guide port diameters were adjusted within design software until the same trephine bur of known diameter interacted with stents from each printer in a consistent and reproducible manner. This allowed examiners to adjust design software diameter settings, taking into consideration each individual printer’s distinctive performance characteristics. Resultant calibration port diameter increases for the Objet260, Form 2, and the Duplicator7 were 0.05 mm, 0.15 mm, and 0.3 mm, respectively.
Stent fabrication: The same STL file used in the introductory TEMS publication for tooth No. 2 was used to print 30 guides from each of the three printers, applying calibration adjustments previously described above (Figure 3B). Objet260 Group: 3DSGs were printed with the Objet260 using proprietary MED610 resin (Stratasys, Eden Prairie, Minnesota). Form 2 Group: 3DSGs were printed with the Form 2 using NextDent SG resin (NextDent, Soesterberg, Netherlands). Duplicator7 Group: 3DSGs were printed with Duplicator7 using NextDent SG resin.
Stent assessment: In order to avoid abrasion of the resin model osteotomy path by the cutting tip of the trephine during repeated insertion into the model osteotomy site, the trephine was measured with digital calipers, and a resin rod precisely replicating the bur diameter was designed and printed. The rod had a 1-mm wide groove at one extent, indicating the proper insertion depth for a clinically acceptable stent.
The two Board-certified endodontists who pioneered the TEMS procedure tested the 3DSGs in the following manner. Each guide was placed on the model and complete seating was verified by inspecting the disto-facial cusp of tooth No. 2 and the incisal edge of tooth No. 9 for intimate stent contact. Finger pressure was applied to the stent, and the rod was inserted through the guide tube. If the rod could be inserted to the full depth of the osteotomy (to the depth indicator groove) with clinically desirable interaction with the guide port, the 3DSG was considered acceptable (Figure 3C). 3DSGs that did not allow for complete insertion of the rod to the depth indicator groove, indicating either an errant osteotomy path or an anomaly in the guide tube diameter, were designated as unacceptable (Figure 3D). Following testing, the rod was measured with digital calipers to ensure its diameter had not been reduced due to multiple passes through the guides. Finally, all 3DSGs were removed from the printed model, and the reference trephine (used to establish resin rod dimensions) was introduced within the lumen. This allowed verification that the interaction of the resin rod and the lumen replicated what would have occurred with the trephine if abrasion of the model were not a consideration. The data was then analyzed using a Fisher’s exact test, and interobserver agreement calculated using Cohen’s Kappa Coefficient.
Results
Part I
All three printers produced objects that deviated from design dimensions, and the null hypothesis was rejected. For all diameters tested, the Objet260 had the lowest mean absolute deviation from the digital design (0.044 mm-0.067 mm) followed by the Form 2 (0.099 mm-0.166 mm). The Duplicator7 had the highest mean absolute difference for all diameters (0.222-0.321 mm). The results of Part I are summarized in Table 2. Post-hoc Tukey’s test determined the mean absolute differences were statistically significant between all three groups for all five diameters tested (p < 0.0001). There was no apparent correlation between diameter size and mean absolute difference for any of the printers.
Part II
All 30 TEMS guides printed by the Objet260 and all 30 TEMS guides printed by the Form 2 successfully guided the rod to ideal osteotomy depth, and the null hypothesis was accepted. In contrast, 22/30 (73.3%) of surgical guides printed by the Duplicator7 allowed insertion of the rod to the ideal osteotomy depth, which was a statistically significant (p < 0.005). There was no interobserver disagreement for any stents tested; therefore kappa = 1. The null hypothesis was rejected. The Duplicator7 3DSG failures all resulted from constrictions or deviations within the stent lumen that would not allow rod penetration. When 3DSGs were removed from the model and tested with the reference trephine, all 3DSG lumens that accommodated the rod also accommodated the trephine, and all 3DSG lumens that failed to accommodate the rod also failed to accommodate the trephine.
Discussion
To our knowledge, this is the first investigation into the accuracy of 3DPs for TEMS.
Previous studies comparing 3DPs for other dental applications have defined outcomes in terms of exact numerical measurements. In one study, there was no significant difference in replica teeth printed from a Fused Deposition Modeling (FDM) printer and a PolyJet device.11 Another study found that orthodontic models made from an SLA device had a statistically significant deviation when compared to PolyJet models only when a specific base geometry was used.13 Endodontic accuracy assessments demonstrated the Form 2’s acceptability for replicating extracted teeth14 and the Objet260’s suitability for guided access,6 but no previous endodontic studies have directly compared printers. There is no gold standard for evaluating these devices.16 Our model sought to simulate an actual clinical scenario documented in the endodontic literature in testing clinically relevant capabilities of the three printers.10 Thus, rather than merely comparing printed product measurements to design measurements, Part II of this study assessed each printer’s ability to consistently produce a clinically acceptable guide.

Based upon dozens of TEMS clinical cases, the authors knew all printed port lumens require a diameter increase over trephine dimensions within design software in order to accommodate the trephine. During post-printing inspection and before use, clinicians must verify that a trephine is able to penetrate the guide tube without resistance. If excessive resistance is encountered, and the trephine is “forced” to pass, it may create an “altered” path with undesirable clinical implications. If a guide tube is excessively larger than the trephine, the osteotomy could deviate from the desired path. The calibration assessment for each printer was utilized to account for differences in print product dimensions for individual printers. If all printers were adjusted with the same tolerance — for example, a 0.15 mm diameter increase over design — one printer could be privileged toward success or doomed to failure more than another based upon its functionality and the specific resin it used. If all printers were adjusted to the absolute mean from Part 1, the interaction of the trephine with the guide port still might not produce adequate clinical performance because of deviations from the mean along a lengthy port lumen.
In the pilot study, we started with values suggested by Part I and adjusted guide ports until desirable interaction with trephines repeatedly occurred, such that the trephine was guided with axial stability for reproduction of a “true” path. After several successive prints from each printer, a clinically oriented tolerance was established. Therefore, Part II assessed each printer’s ability to consistently reproduce clinically acceptable stents utilizing tolerances established in the pilot study.
Like the costly Objet260, the affordable Form 2 showed consistency in printing clinically acceptable 3DSGs for use in TEMS. Although the Duplicator7 produced clinically acceptable stents in the majority of cases, 26.7% of stents had a constricted lumen diameter precluding full insertion of the rod. These stents would not have had clinical utility. Although our experimental model would suggest that all acceptable stents would produce desirable clinical results in vivo, it is impossible to conclude this absolute with certainty.
The results of Part I align well with results in Part II; the standard deviation for the 6.0 mm diameter hole was much higher for the Duplicator7 (0.058 mm) than it was for either the Connex 3 (0.021 mm) or the Form 2 (0.035 mm). This data predicted the lack of consistency of the Duplicator7 seen in Part II.
“Off the box” printer specifications such as XY axis and layer thickness cannot be used for side-by-side comparison of printers because they do not account for a multitude of factors that influence the accuracy of the final product. Potential inaccuracies during stent fabrication can be introduced during data acquisition, design, and fabrication.16,17 Product geometry, orientation, and even resin color may impact the quality of the printed product.18 This study could not control for effects of different resins as manufacturer requirements precluded use of the same resin in each printer. This experimental model shows a process that any lab or individual clinic would need to go through after acquiring a printer, in order to understand its performance characteristics. Other experimental models that merely report on dimensional conformity of object to design do not approach the subject of clinical utility. These results should be interpreted narrowly, as an indication of performance consistency of these three printers in producing stents for TEMS. Indeed, widespread generalization of these data to all PolyJet, SLA, or DLP technical-type printer classifications across a wide array of dental applications is not warranted.
Conclusions
Safe and effective TEMS relies upon an accurate 3DSG that can accommodate a trephine bur along a precisely designed pathway. A 3DSG that alters the path of the trephine or that altogether fails to accommodate the trephine must be discarded with subsequent delay or alteration in treatment. The Objet260 and Form 2 produced objects with low dimensional deviations from digital design.
Clinical Implications
The Objet260 and the Form 2 consistently produced clinically acceptable 3DSGs and represent viable 3D printing options for TEMS applications.
Disclaimer
The views expressed are those of the authors and do not reflect the official views or policy of the Department of Defense or its Components or the Uniformed Services University of the Health Sciences.
Acknowledgments
Special acknowledgments to Mr. Daniel Sierra and Mr. James Pizzini at Air Force Postgraduate Medical CAD/CAM Lab.
After reading this study on Targeted Endodontic Microsurgery, check out this book review for Microsurgical Endodontics by Drs. Dr. Bertrand Khayat and Dr. Guillaume Jouanny.
https://endopracticeus.com/products/microsurgical-endodontics/
- Lee SJ, Jung IY, Lee CY, Choi SY, Kum KY. Clinical application of computer‐aided rapid prototyping for tooth transplantation. Dent Traumatol. 2001;17(3):114-119.
- Pinsky, HM, Champleboux, G, Sarment, DP. Periapical surgery using CAD/CAM guidance: preclinical results. J Endod. 2007;33(2):148-151.
- Meer WJ, Vissink A, Ng YL, Gulabivala K. 3D computer aided treatment planning in endodontics. J Dent. 2016;45:67-72.
- Strbac GD, Schnappauf A, Giannis K, et al. Guided autotransplantation of teeth: a novel method using virtually planned 3-dimensional templates. J Endod. 2016;42:1844-1850.
- Strbac GD, Schnappauf A, Giannis K, Moritz, A, Ulm C. Guided modern endodontic surgery: a novel approach for guided osteotomy and root resection. J Endod. 2017;43(3):496-501.
- Connert T, Zehnder MS, Weiger R, Kühl S, Krastl G. Microguided Endodontics: Accuracy of a Miniaturized Technique for Apically Extended Access Cavity Preparation in Anterior Teeth. J Endod. 2017;43(5):787-790.
- Anderson J, Wealleans J, Ray J. Endodontic applications of 3D printing. Int Endod J. 2018;51(9):1005-1018.
- Kim GB, Lee S, Kim H, et al. Three-Dimensional Printing: Basic Principles and Applications in Medicine and Radiology. Korean J Radiol. 2016;17(2):182-197.
- Schoffer F. How expiring patents are ushering in the next generation of 3D printing. TechCrunch.com. 2016. https://techcrunch.com/2016/05/15/how-expiring-patents-are-ushering-in-the-next-generation-of-3d-printing/. Accessed on January 27, 2020.
- Giacomino CM, Ray JJ, Wealleans JA. Targeted endodontic microsurgery: A novel approach to anatomically challenging scenarios using 3-dimensional-printed guides and trephine burs — a report of 3 cases. J Endod. 2018;44(4):671-677.
- Lee KY, Cho JW, Chang NY et al. Accuracy of three-dimensional printing for manufacturing replica teeth. Korean J Orthod. 2015;45(5):217-225.
- Favero CS, English JD, Cozad BE, et al. Effect of print layer height and printer type on the accuracy of 3-dimensional printed orthodontic models. Am J Orthod Dentofacial Orthop. 2017;152(4):557-565.
- Camardella LT, de Vasconcellos Vilella O, Breuning H. Accuracy of printed dental models made with 2 prototype technologies and different designs of model bases. Am J Orthod Dentofacial Orthop. 2017;151(6):1178-1187.
- Reymus M, Fotiadou C, Kessler K, et al. 3D printed replicas for endodontic education. Int Endod J. 2019;52(1):123-130.
- Cristache CM, Gurbanescu S. Accuracy Evaluation of a Stereolithographic Surgical Template for Dental Implant Insertion Using 3D Superimposition Protocol. Int J Dent. 2017.
- Block MS, Chandler C. Computed tomography – guided surgery: complications associated with scanning, processing, surgery, and prosthetics. J Oral Maxillofac Surg. 2009;67(Suppl 11):13-22.
- D’haese J, Van De Velde T, Komiyama A, Hultin M, De Bruyn H. Accuracy and complications using computer-designed stereolithographic surgical guides for oral rehabilitation by means of dental implants: a review of the literature. Clin Implant Dent Relat Res. 2012;14(3):321-335.
- Olszewski R, Szymor P, Kozakiewicz M. Accuracy of three-dimensional, paper-based models generated using a low-cost, three-dimensional printer. J Craniomaxillofac Surg. 2014;42(8):1847-1852.
Stay Relevant With Endodontic Practice US
Join our email list for CE courses and webinars, articles and more..